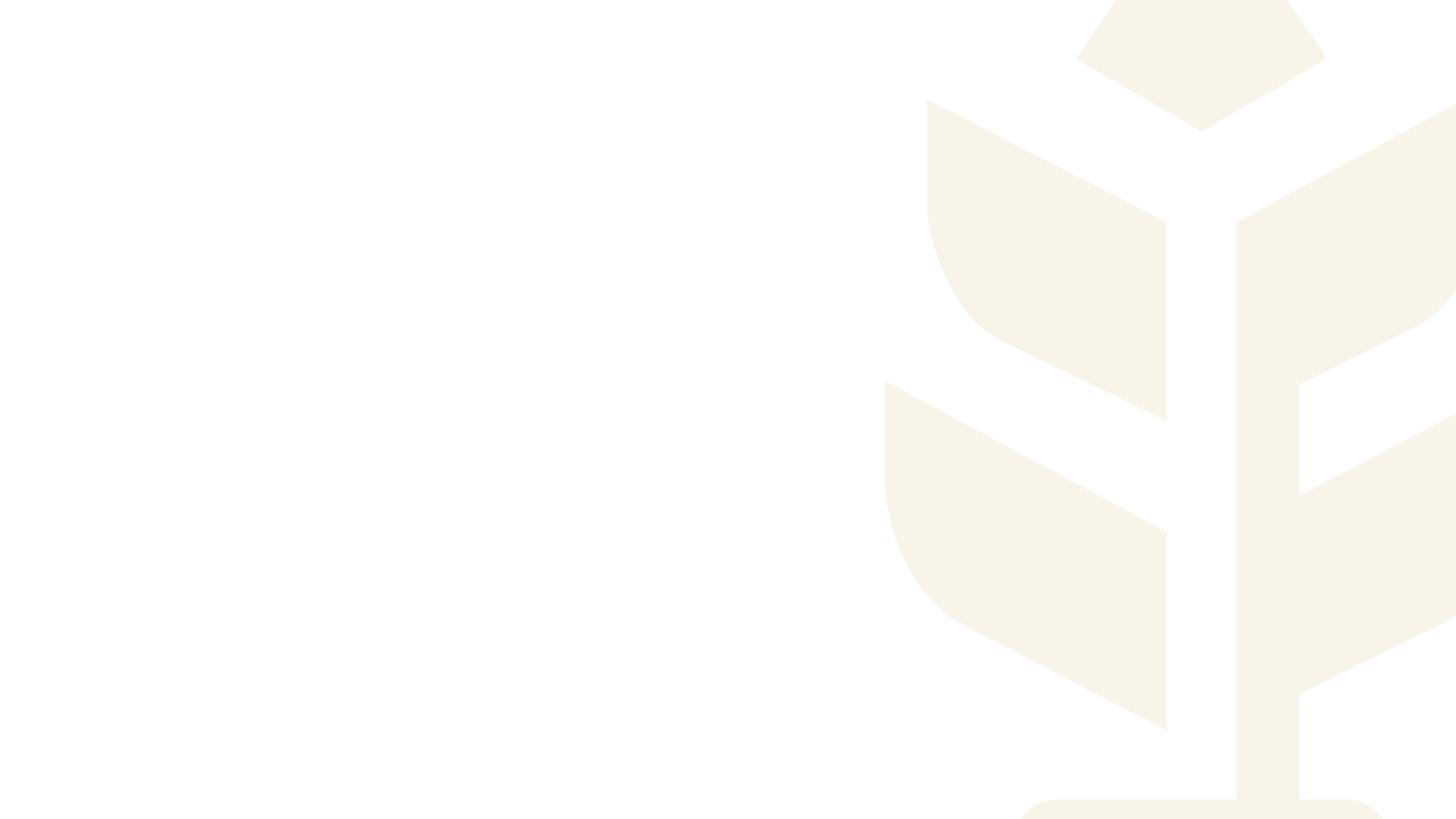
Core knowledge
FAQs
General Advanced Nuclear Technology
-
Advanced nuclear energy refers to a new generation of nuclear reactor technologies designed to improve the safety and efficiency of traditional nuclear power. These reactors use innovative designs, materials, and fuel cycles, such as Small Modular Reactors (SMRs), Micro Modular Reactors (MMR), molten salt reactors, and fast reactors, to address challenges faced by older nuclear plants.
-
Advanced nuclear reactors differ from traditional reactors in several ways:
Safety: Many advanced designs incorporate passive safety features that can automatically shut down the reactor in case of emergencies without human intervention.
Efficiency: New reactor designs aim to use fuel more efficiently, often extracting more energy from the same amount of nuclear material.
Waste Reduction: Some advanced technologies have the potential to recycle nuclear waste or produce less long-lived radioactive waste than traditional reactors.
Modularity: Advanced reactors offer a more flexible deployment, allowing for scalable power production and reduced construction times.
-
Yes. Advanced nuclear reactors are designed with safety as a top priority. Many utilize passive safety systems that operate without human control or external power, reducing the risk of accidents. Some designs even have inherent safety features that prevent meltdowns by relying on natural physical processes, such as gravity and convection, to cool the reactor in the event of a malfunction.
-
Nuclear energy provides baseload power, meaning it can operate continuously and provide a stable energy supply, while solar and wind are intermittent and depend on weather conditions. Combining advanced nuclear with renewables can create a resilient and diverse energy grid that meets energy demand at all times.
-
Advanced nuclear technologies are being designed with cost reduction in mind. Most advanced reactors can be built in factories, reducing construction costs and time. Additionally, new reactor designs aim to operate more efficiently and require less frequent refueling, which lowers operational costs. As these technologies mature, the cost of advanced nuclear is expected to become competitive with other forms of clean energy once we can move from first-of-a-kind costs to nth-of-a-kind costs.
-
Advanced nuclear is expected to play a significant role in the global energy mix, providing reliable, dispatchable, carbon-free energy. Its ability to offer a steady supply of electricity makes it a vital part of future energy strategies, particularly as energy demand grows.
-
Advanced nuclear reactors are expected to be commercially available by the late 2020s or early 2030s. Many countries and companies are investing heavily in nuclear innovation, and the timeline for deployment is shortening as regulatory processes adapt and new technologies become ready for market.
-
Advanced nuclear energy has a minimal environmental impact. It produces no greenhouse gas emissions during operation and uses significantly less land and water than many renewable energy sources. While there are still challenges associated with nuclear waste, advanced reactors are designed to minimize waste production and improve waste management practices, further reducing their environmental footprint.
-
Developing a workforce capable of supporting advanced nuclear technologies involves a multifaceted approach. Key steps include establishing comprehensive education and training programs at universities and vocational schools and fostering industry partnerships for real-world experience. Specific workforce opportunities include nuclear engineering, reactor design, component manufacturing, supply chain, logistics, fuel management, construction, and reactor operations and support.
Shepherd Power is committed to supporting workforce development by partnering with educational institutions to align curricula with industry needs, offering internships and apprenticeships for hands-on experience, and providing mentorship programs as the industry develops.
-
SMR stands for Small Modular Reactor. This type of nuclear reactor is smaller in size than traditional nuclear reactors and is designed to be manufactured at a plant and transported to a site for assembly. The modular aspect allows for components to be added incrementally to match increasing energy demands. SMRs are seen as a promising technology because they offer several benefits:
Enhanced Safety: Smaller size and advanced safety features can result in lower risk.
Cost Efficiency: Lower initial capital investment compared to larger reactors, theoretically.
Flexibility: Can be deployed in remote locations and integrated with renewable energy sources.
Scalability: Can be scaled up as needed by adding more modules.
MMR stands for Micro Modular Reactor. This type of nuclear reactor is even smaller than SMRs and is designed to provide a very localized power supply, typically for remote or off-grid locations. MMRs are characterized by:
Compact Size: Typically producing less than 15 megawatts of electrical power.
Portability: Can be transported via road or waterway and set up quickly.
Autonomy: Designed to operate with minimal human intervention and maintenance.
Rapid Deployment: Short construction times due to their small size and modular design.
LWR stands for Light Water Reactor. This is the most common type of large nuclear reactor used around the world for electricity generation. LWRs use normal water (as opposed to heavy water) as both a coolant and a neutron moderator. There are two main types of LWRs:
Pressurized Water Reactors (PWRs): In these reactors, water is kept under high pressure to prevent it from boiling, and heat is transferred to a secondary water system via a heat exchanger to produce steam that drives a turbine.
Boiling Water Reactors (BWRs): In these reactors, water is allowed to boil inside the reactor vessel, and the generated steam directly drives the turbine.
Reactors
-
Nuclear reactors have evolved over time, and the designs of Generation IV reactors reflect advancements in technology, safety, and efficiency that were not available or fully understood when earlier reactors were built. Initially, nuclear reactor designs, such as those from the Generation I and II eras, were developed rapidly to meet the urgent demands for energy and military applications during the mid-20th century. These early designs focused on proven technologies and materials that were available at the time.
Several factors have contributed to the evolution of Generation IV reactors:
Technological Advances: Innovations in materials science, computer modeling, and reactor physics have enabled the development of more advanced and safer reactor designs.
Safety Enhancements: Lessons learned from past nuclear incidents and a deeper understanding of reactor behavior under various conditions have driven the incorporation of enhanced safety features in modern designs.
Efficiency and Sustainability: Generation IV reactors aim to improve fuel efficiency, reduce nuclear waste, and utilize alternative fuel cycles, addressing concerns about resource sustainability and waste management.
Environmental and Economic Considerations: Modern reactors are designed to be more environmentally friendly and economically viable, with features such as reduced greenhouse gas emissions and lower operational costs.
Regulatory and Public Perception: Stricter regulatory requirements and increased public scrutiny have necessitated more robust safety and environmental performance in new reactor designs.
The progression to Generation IV reactors is a result of continuous improvement and adaptation to new scientific knowledge, technological capabilities, and societal needs.
-
These reactors are designed to have an operational lifespan of 5 to 12 years per reactor core, during which they will consistently produce energy. After this period, the core can be replaced or refueled to extend the reactor's life and continue its energy production, ensuring a reliable and long-term power solution.
-
Yes, advanced reactors generate waste heat that can be recovered and utilized. This waste heat can be harnessed for various applications, such as district heating, industrial processes, and desalination. By effectively capturing and using the waste heat, advanced reactors can improve overall energy efficiency and provide additional economic and environmental benefits.
Regulatory
-
Shepherd Power is leading Project Hotshot, a collaborative effort with several stakeholders, including the Nuclear Energy Institute (NEI), that is focused on modernizing regulations to enable fast, scalable deployment of advanced reactors without sacrificing safety. This initiative addresses 31 key areas where the existing regulatory framework may not appropriately account for the benefits of advanced reactor technologies, including environmental reviews, construction and operational oversight, as well as testing manufactured reactors in a factory setting. Our work has focused attention on 180-day new reactor licensing timelines, lower Nuclear Regulatory Commission (NRC) fees, and risk-informed regulations that cut costs while maintaining safety.
-
For advanced nuclear to be cost-competitive with traditional energy sources, key regulatory reforms must reduce licensing complexity, lower costs, and enable faster deployment. The current NRC framework was built for large, gigawatt-scale nuclear plants. Implementation of this framework for advanced reactors could lead to unnecessary delays and costs that make advanced reactors uneconomical without a corresponding increase in safety. Addressing these barriers is critical to scaling production, cutting regulatory overhead, and ensuring advanced reactors can be deployed at commercially viable prices.
Key Regulatory Changes for Commercial Viability
Faster, Lower-Cost Licensing
Current Issue: The licensing process is slow and expensive, requiring years of NRC review and millions in fees, even for reactors designed to be inherently safe and prefabricated.
Needed Reform: Implement a 180-day licensing pathway, capping NRC fees at less than 1% of total capital costs to ensure regulatory expenses don’t make projects uneconomical.
Streamlined Environmental Reviews
Current Issue: The NRC develops and issues Environmental Impact Statements (EISs) for every new reactor application to comply with the requirements of the National Environmental Policy Act (NEPA). These EISs are multi-year efforts that may not align with the environmental impacts of new advanced reactors.
Needed Reform: The NRC should recognize the minimal environmental impacts associated with advanced reactor technologies by using Environmental Assessments or Categorical Exclusions instead of EISs to streamline environmental reviews while ensuring compliance with NEPA.
Sensible Security and Emergency Planning
Current Issue: Security and emergency response rules assume large reactor facilities with on-site staff, imposing armed security and evacuation zones, even for low-risk microreactors in remote locations.
Needed Reform: Implement a risk-informed security approach that eliminates armed response requirements for sites where the worst-case accident poses minimal offsite risk.
Flexible Siting and Construction Requirements
Current Issue: Advanced reactors don’t need the same siting constraints as large plants, yet they must undergo lengthy geological and hydrological studies before site approval. Existing federal datasets (National Oceanic and Atmospheric Administration, US Geological Survey, etc.) could also be used to gather relevant siting information while ensuring that site safety will not be compromised.
Needed Reform: Enable pre-licensed factory-built reactors with a national siting framework, allowing for modular deployment without redundant site-specific reviews. Permitting the use of existing datasets to characterize sites would also streamline site characterization efforts.
Expanded Supply Chain Access
Current Issue: NRC regulations restrict the manufacturing of certain nuclear components to a small number of specialized vendors, driving up costs and limiting scalability.
Needed Reform: Allow vendors using quality management standards (e.g., ISO 9001) comparable to those used in the nuclear industry to manufacture components, broadening the supply chain and lowering production costs.
By implementing these targeted regulatory changes, advanced nuclear can move from a slow, one-off licensing model to a scalable, repeatable process that drives down costs and accelerates deployment. These reforms will enable advanced reactors to compete with diesel and natural gas for industrial, off-grid, and continuous power applications, making carbon-free nuclear energy truly economical.
Shepherd Power
-
Shepherd Power is building the foundation for rapid, scalable advanced reactor deployment, and we’re ready to discuss how they fit into your energy strategy. While the first commercial units are expected to be available by the early 2030s, we are actively working to clear regulatory hurdles, develop deployment pathways, and align supply chains to make advanced reactors a turnkey energy solution when the technology is ready.
If you’re serious about securing reliable power, now is the time to start the conversation. We can walk you through site selection, regulatory planning, and operational integration so that when the first units are ready, you’re first in line. Let’s connect and map out your path to scalable, resilient energy.